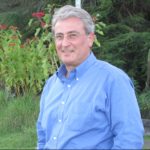
An Interview with Razmik Mirzoyan
11 March 2015
MAGIC project leader at the Max-Planck-Institute for Physics, Munich, and spokesperson of the MAGIC Collaboration.
You are Project Leader and Spokesperson for the MAGIC collaboration. What are the major goals and research directions of the collaboration?
The Imaging Atmospheric Cherenkov Technique (IACT) has made giant steps – in fact it became a powerful new branch of astrophysics. With the discovery of very high energy (VHE) gamma rays from the Crab Nebula in 1989, the Whipple team laid the foundation for the new science. Other telescope installations, like HEGRA and CANGAROO, belonging to the so-called 2nd generation IACTs, very soon started their own search of gamma ray sources. Although some 10 sources were discovered by the year 2000, still all of those detections were made above the energy threshold of ~300 GeV.
Already in 1995, at a few conferences, we had presented the concrete concept of the 17m diameter MAGIC telescope. Shortly before we had understood that the energy threshold of detection (Ethreshold) was inversely proportional to the area of the mirror (1/√Amirror) and not the square root of the area as is the case for non-imaging telescopes, showing thus that one can build a moderately large diameter telescope for operating in the domain of sub-100 GeV energies.
The MAGIC design was aiming to go towards the sub- 100 GeV energy range down to 30-40 GeV, into the last decade, “terra incognita” of the electromagnetic emission spectrum, traditionally considered as the classical domain of satellite-born instruments. MAGIC people were anticipating finding new classes of sources such as, for example, the pulsars. Also, because of strong absorption of TeV gamma-rays by the extragalactic background light, MAGIC was aiming to measure sources in the sub-100 GeV range, where the universe is much more transparent to gamma rays and one can search for powerful sources located at large redshifts.
We have spent a lot of effort planning the telescope to be light-weight, for fast repositioning of the telescope in response to the alerts os satellites spotting Gamma Ray Bursts, trying to measure them still in the prompt phase of the emission. For that purpose we built the reflector of the telescope from reinforced carbon fibre, which is several times lighter than the steel but has a comparable stiffness and very low thermal expansion. For the same reason we introduced the concept of the Active Mirror Control to the VHE Gamma Astrophysics, allowing one to counteract the deformations of the reflector due to varying gravitational loads when tracking sources.
Today we are happy that our expectations were largely fulfilled. In 2008 we found the first pulsed signal from the Crab pulsar at energies above 25 GeV, for the first time creating a bridge between satellite detectors and IACTs. Recently we found that the spectrum of the pulsating gammas from Crab is extending till ~2 TeV, i.e. that an object of a ~ 10km size can accelerate electrons to a gamma factor of ~ 107. By using the data of the Fermi satellite and of MAGIC we measured very precisely the peak position of ~ 55 GeV of the Inverse Compton emission of the Crab Nebula. Recently, via gravitational lensing, we found the new source S3 0218 located at a redshift of almost 1 (z=0.944). The list of highly interesting results, both of extragalactic and galactic origin, is really long and it is progressively updated with newer results.
Both ETE and Hamamatsu went through several development iterations, consequently improving the key PMT parameters, one at a time. Left: two 8-dynode PMTs from ETE of intermediate types D872 and D569/2SA, clear input window. Right: PMT R12992-100 (7-dynodes) and R11920-100 (8-dynode) from Hamamatsu, both of mat window type. The final 7-dynode PMTs from both companies will be compatible in pin, size and window type.
Could you give a brief overview on light sensor technology used in astroparticle physics?
The classical photomultiplier tubes (PMTs) are the main detectors used as light sensors in astroparticle physics experiments. In a dedicated two-stage development program (at first for MAGIC and since 2010 for the CTA collaboration), in tandem with industrial partners Hamamatsu (Japan) and Electron Tubes Enterprises (England), we succeeded to significantly improve the performance of PMTs. Today we arrived at 1.5 inch size commercial PMTs for the CTA project, which have an average peak quantum efficiency (QE) of ~40%, photo electron collection efficiency in excess of 95%, and about 50 times reduced after-pulsing. Now these are the best PMTs worldwide. We anticipate that these novel technologies will be applied also to the large size PMTs, much needed in neutrino experiments.
Silicon photomultipliers (SiPMs) emerged as novel photo sensors some 15 years ago. In 2003 we suggested to use them in astroparticle physics experiments, initially in EUSO and in MAGIC, and actively participated in their development. Some seven years ago we started constructing realistic pixels for IACTs. This initiative has been taken by our colleagues from the FACT collaboration that succeeded constructing a full-scale operational camera. My colleagues from the CTA collaboration are planning to use SiPMs for the cameras of the so-called small size telescopes (~4m diameter class) as well as for the mid-size Schwarzschild-Couder telescope. The parameters of SiPMs are still improving, every 6-8 months we face novel and better products from several manufacturers. For the time being the best SiPM samples come close to the parameters of the best PMTs. The SiPMs have the potential to achieve a photon detection efficiency (PDE) of ~60-65% at an acceptable low cross-talk level of a few percent; this may be within reach within the next couple of years. The SiPMs have a small size of typically 3mm×3mm or 6mm×6mm. The limitation on the size is due to the requested fast timing of a few nanoseconds, as well as, due to the increasing with size gain and cross-talk. Currently a few groups are trying to build close-packed matrixes of SiPMs for emulating PMTs of 1-1.5 inch size, but the results still need to be carefully evaluated.
Since a few years several manufacturers offer integrated matrixes of SiPMs. The final goal will be to arrive at optimized in performance, ns-fast matrixes, which will include on the rear side the readout of the measured charge, similar like today’s CCD and CMOS cameras do. Given these, one can assemble, as Lego, high-resolution imaging devices of arbitrary size and shape. These will revolutionize not only the physics experiments but also the imaging devices in medicine and will find extremely wide applications everywhere.
What are the main innovations/technological improvements in the use of light sensors in astroparticle physics?
Since the mid 1960’s the main parameters of PMTs were stagnating for about 40 years. The main innovation in light sensors in the past 10 years are related to the enhancement of their photon detection efficiency and the suppression of the unwanted after-pulsing in PMTs and cross-talk and after-pulsing in SiPMs. About 10 years ago the typical PMT had a peak QE of ~25%, photo-electron collection efficiency of 80% and an after-pulsing level of 0.5% for the set threshold of 4 photo electrons. Today we have PMTs with peak QE in excess of 40%, photo electron collection efficiency of 95-98% and an after-pulsing below 0.01%.
A faster improvement can be observed for SiPMs. While SiPMs from Hamamatsu, named MPPC (multi-pixel photon counter), were showing a peak PDE of 30-35% ~5 years ago, today there are devices with a peak PDE of ~45%. But for the majority of experiments the PDE of SiPMs alone is not a very meaningful parameter; one needs to consider the cross-talk and the after-pulsing levels for understanding the limitations in the aimed application. Today SiPM samples from several manufacturers show cross-talk at a several percent level; this is to be compared with their one order of magnitude higher level in the relatively recent past.
Today SiPM cost is becoming progressively low. This is unfortunately not the case for the readout systems. For planning an astroparticle physics detector one needs to carefully consider costs. Even with comparable photon detection efficiencies of SiPMs and PMTs, simply substituting the PMTs by assembled matrixes of many SiPMs may not necessarily offer an optimal solution. In addition, the assembled matrixes of SiPMs may not be as fast as the PMTs; also, it is well known that even a single SiPM has a significantly higher noise rate than a PMT. But as I mentioned earlier, the SiPM parameters are still improving and when their PDE will exceed that of PMTs by ~50%, they may become very strong competitors for PMTs, albeit only for small sensor size applications.
What are the technological advances in ground-based gamma-ray astronomy? And what are the upcoming plans?
The technological advances in ground-based gamma-ray astronomy are multi-fold, they can be observed taking as an example the (differing in size and performance) telescopes of the CTA collaboration:
- Use of novel technologies for mirror production providing longevity (mirrors currently used for IACTs are strongly deteriorating after ~3 years).
- The Active Mirror Control System finds wide application in large-size telescopes. This provides optimal focusing of telescopes independent on observation angles.
- Novel readout systems in the imaging cameras provide an order of magnitude lower cost compared to what was used just a few years ago.
- We are moving towards sealed, temperature-controlled imaging cameras; this is necessary for the desired robotic operation of the telescopes in the future and for the reduced maintenance of multiple telescopes in high-mountain altitude or desert locations.
- Double-mirror telescope designs are paving the road. They are using SiPM-based cameras, offer a compact design for a wide field of view and promiss an improved angular resolution. The main challenges here are related to the secondary mirror which needs high precision both in quality and fixation in the structure. The first ASTRI telescope of the Italian INAF was inaugurated in Sicily in September 2014. Probably within a time scale of a year we will have results from the first measurements. The main problem with the double-mirror designs, compared to simple prime focus telescopes, is their high cost.
Speaking about future plans, I believe that the last word in the scene of light sensors is not yet said, not only the SiPM but also the PMT parameters can still be improved significantly. I am part of a small community of photo-cathode-related specialists-enthusiasts who are dreaming about a PMT with peak QE as high as maybe 70% (for the time being we have few samples of 45-47%). For me, this is essentially linked to the question of availability of financial resources and coordinated efforts of a few years.
You are heading a Russian-German collaboration at Tunka Valley, operating a large-scale experiment studying cosmic rays and gamma-rays. Could you say something about this project and its expected outcomes?
The experiment dubbed as TAIGA in Tunka valley, near the lake Baikal, is really interesting. Currently TAIGA has an operational 185 station TUNKA open-air-integrating Cherenkov detectors. An underground muon detector is under construction for vetoing muons. The experiment is hosting an array of radio detectors from our colleagues from Karlsruhe.
Our idea is to try to operate a single small-size, wide-angle imaging Cherenkov telescope together with the wide-angle integrating Cherenkov detectors (dubbed HiSCORE), which (initially) will be spread over a distance of ~600 m from the telescope. This combination can be considered as a “cell” of a future large-size instrument. Since some time now, the concept of HiSCORE, along with that of the imaging telescope, is pursued by my colleagues from the University of Hamburg and DESY (from Hamburg and Zeuthen locations), together with experienced research teams from MEPhi, SINP MSU, ISU and JINR Dubna in Russia. The combination of a low-coat wide-angle imaging Cherenkov telescope with the so-called HiSCORE detectors, spread over large distances, has the promise to offer very large collection area for measuring the spectra of tens of TeV to hundreds of TeV gamma-ray sources (so called PeVatrons). Compared to planned alternatives, this is a really low-cost detector. We will try to prove this principle in the coming years. Some 30 HiSCORE stations are now operational and the first imaging telescope is under construction.
Further reading:
HEGRA: The High Energy Gamma Ray Astronomy experiment was located on La Palma (Canary Islands) at a height of 2200 m. It consisted of several detector types for observing secondary particles or light from cosmic ray particle cascades in the atmosphere, in the energy range 1012 to 1016 eV. The strongest detector of HEGRA was the array of six ~4m class IACTs. HEGRA was taking data between 1987 and 2002.
CANGAROO III: Collaboration of Australia and Nippon (Japan) for a Gamma-Ray Observatory in the Outback. The experiment uses an array of 4 imaging atmospheric Cherenkov telescopes in Australia.
CTA: Cherenkov Telescope Array is an initiative to build the next generation ground-based very high energy gamma-ray instrument. CTA is included in the 2008 roadmap of the European Strategy Forum on Research Infrastructures (ESFRI), is one of the “Magnificent Seven” of the European strategy for astroparticle physics published by ASPERA, and highly ranked in the “strategic plan for European astronomy” of ASTRONET.
A.M. Hillas, “Evolution of ground-based gamma-ray astronomy from the early days to the Cherenkov Telescope Arrays”, Astropart. Phys., 43, (2013), 19-43
E. Lorenz, R. Wagner, “Very High Energy Gamma-Ray Astronomy. A 23 Year Success Story in High Energy Astroparticle Physics”, Europ. Phys. J. H, 37, 3 (2012) 459-513
R. Mirzoyan, “Brief History of Ground-Based very High Energy Gamma-Ray Astrophysics with Atmospheric Air Cherenkov Telescopes”, Astropart. Phys., 53 (2014) 91-99
Dr. Razmik Mirzoayn is permanent staff member at the Max Planck Institute for Physics in Munich, the current Spokesperson of the MAGIC collaboration and the Principal Investigator and Spokesperson of the TAIGA collaboration. He is honorary professor and head of the laboratory “Multi-TeV Energy Gamma-Ray Astronomy” at the Irkutsk State University in Russia. He recently became foreign member of the National Academy of Sciences of the Republic of Armenia.
Dr Mirzoyan is one of the founders of the HEGRA IACT system, one of the founders of the MAGIC telescopes system, and has strongly contributed to CTA, especially in the design of the imaging cameras. He has strong interest in light sensors, in developing PMTs, HPDs and SiPMs.
He received his PhD (1985) from the Lebedev Physics Institute of the Academy of Sciences of USSR in Moscow. He is author/co-author of over 500 publications (over 200 in peer-reviewed journals) and he is co-authoring six patents.
Submitted by Eleni Chatzichristou
APPEC Communications Officer